The lab report below was submitted as part of the coursework for
CM3291 Advanced Experiments in Organic and Inorganic Chemistry. Please do not plagiarise from it as
plagiarism might land you into trouble with your university. Do note
that my report is well-circulated online and many of my juniors have
received soft copies of it. Hence, please exercise prudence while
referring to it and, if necessary, cite this webpage.

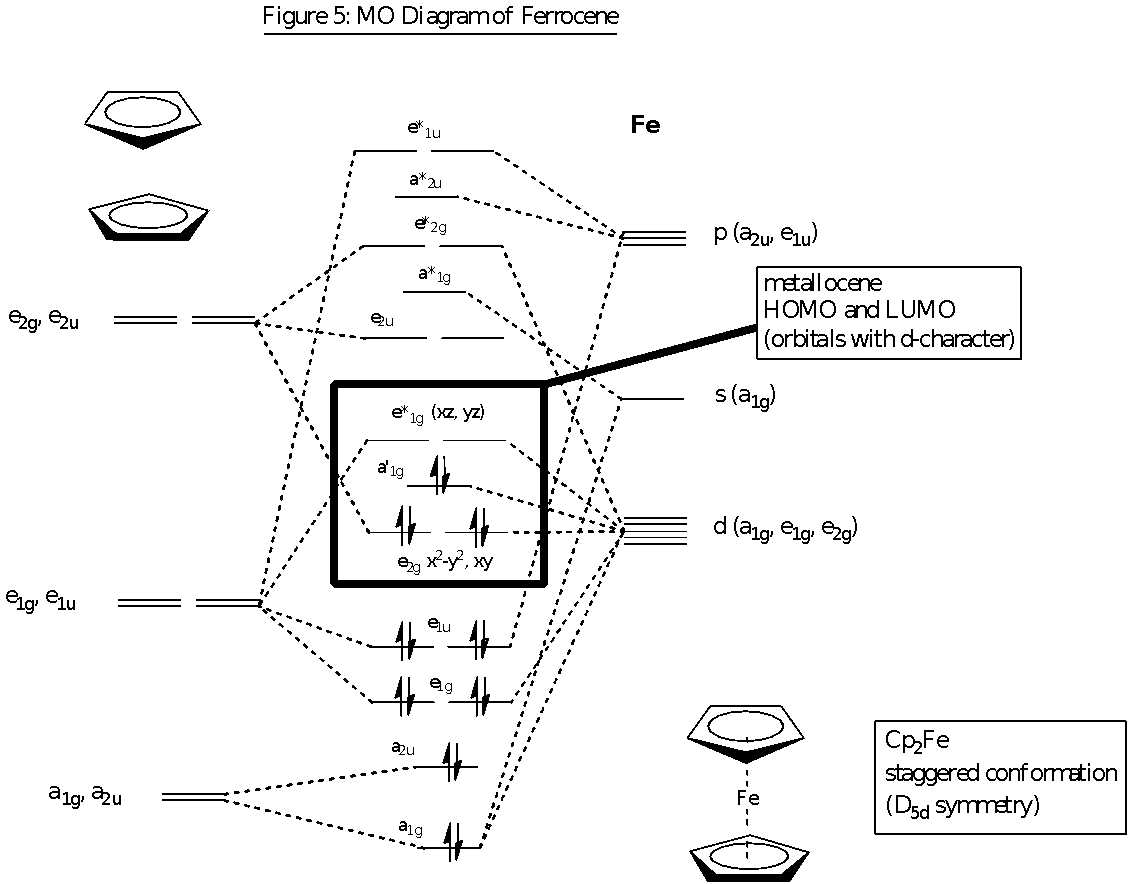
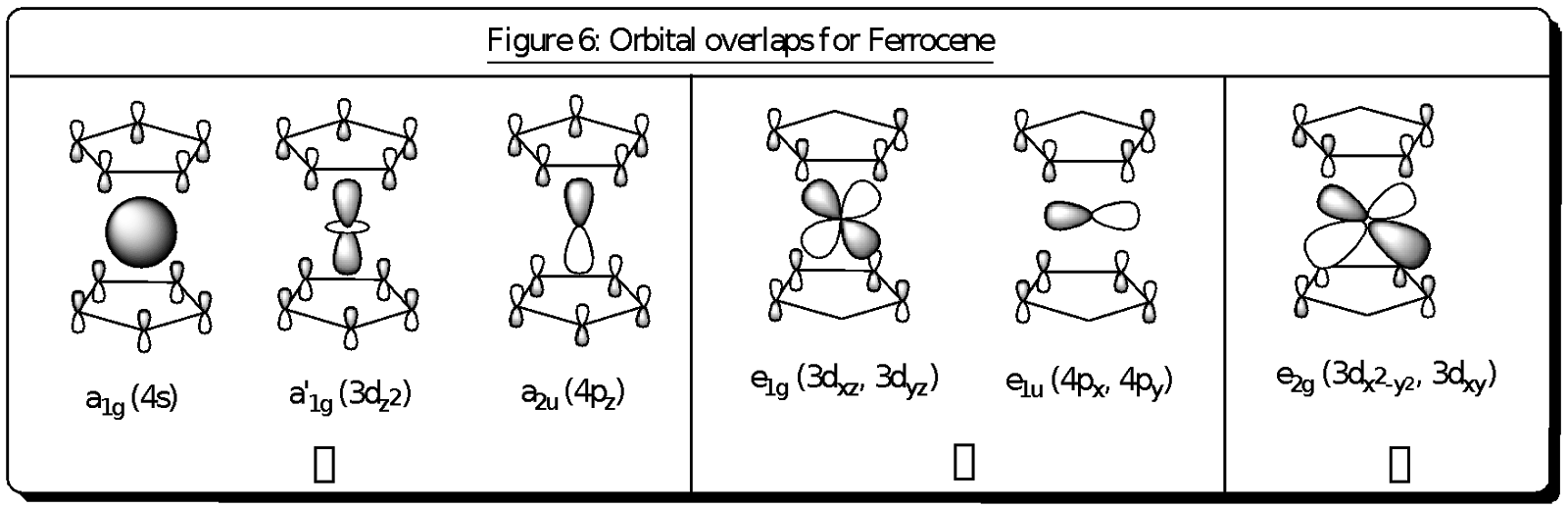
Meanwhile, the other two peaks in the spectrum at 1.5 ppm and 7.24 ppm are due to H2O and CHCl3. The solvent used in the NMR is CDCl3 but this solvent is not entirely deuterated, there is still a small percentage of solvent present as CHCl3 which gives a characteristic peak at 7.24 ppm. The other peaks in the spectrum are calibrated with respect to this reference signal. The peak at 1.50 ppm is due to H2O present which means that the ferrocene is not completely dry and there were traces of water present in the sample tested for NMR. The water present presumably comes from water vapour condensing on the product during the sublimation process.
Aim
This experiment aims to synthesize ferrocene from 1,3-cyclopentadiene and iron (II) chloride under basic conditions. The product is to be purified using sublimation, after which its melting point is determined and characterised with IR spectroscopy.
Results & Calculations
Synthesis of Ferrocene
2 C5H6 + 8 KOH + FeCl2.4H2O -> Fe(C5H5)2 + KCl + 6 KOH.H2O
Mass of KOH used: 4.0081 g
Molar mass of KOH: 39.10 + 16.00 + 1.01 = 56.11 g mol-1
No. of moles of KOH used: 4.0081 / 56.11 = 71.43 mmol
Vol of C5H6 used: 1.0 ml
Density of C5H6: 0.786 g ml-1
Mass of C5H6 used: 1.0 x 0.786 = 0.786 g
Molar mass of C5H6: 5 x 12.01 + 6 x 1.01 = 66.11 g mol-1
No. of moles of C5H6 used: 0.786 / 66.11 = 11.889 mmol
Mass of FeCl2.4H2O used: 1.3021 g
Molar mass of FeCl2.4H2O: 55.85 + 2 x 35.45 + 4 x (2 x 1.01 + 16.00) = 198.83 g mol-1
No. of moles of FeCl2.4H2O used: 1.3021 / 198.83 = 6.548 mmol
The mole ratio of C5H6 to KOH to FeCl2.4H2O is 2:8:1. As 11.889 mmol of C5H6 required 47.556 mmol of KOH and 5.945 mmol of FeCl2.4H2O, C5H6 is the limiting reagent.
Expected no. of moles of Fe(C5H5)2: 5.945 mmol
Molar mass of Fe(C5H5)2: 55.85 + 2 x (5 x 12.01 + 5 x 1.01) = 186.05 g mol-1
Theoretical mass of Fe(C5H5)2 to be obtained: 5.945 x 10-3 x 186.05 = 1.106 g
Actual mass of Fe(C5H5)2 obtained: 0.3622 g
Percentage yield of Fe(C5H5)2: 0.3622 / 1.1060 x 100% = 32.7%
Melting point range of Fe(C5H5)2: 172.80 – 173.90 oC
IR Spectrum Analysis of Fe(C5H5)2
Experimental
|
Literature
|
Intensity of peak
|
Vibrational mode
|
Wavenumber (cm-1)
| |||
3462.4
|
3440.70
|
Weak and broad
|
O-H stretch
|
3094.0
|
3094.34
|
Weak
|
sp2 C-H stretch
|
1637.1
|
1636.31
|
Weak and broad
|
Aromatic overtones
|
1407.0, 1105.6
|
1407.98, 1105.73
|
Medium and sharp, strong and sharp
|
C=C stretch
|
1000.7
|
1000.96
|
Strong and sharp
|
sp2 C-H in-plane bending
|
815.5, 786.00
|
816.03, 787.00
|
Strong and sharp, medium and sharp
|
sp2 C-H OOP bending
|
490.2
|
490.86
|
Strong and sharp
|
Cp tilt
|
475.6
|
476.28
|
Strong and sharp
|
Fe – Cp stretch (anti-sym)
|
Discussion
A: The cracking of dicycopentadiene
In the synthesis of ferrocene, it was necessary to first prepare 1,3-cyclopentadiene. However, the cyclopentadiene was not stable at room temperature because it can readily form the dimer dicyclopentadiene by a Diels-Alder reaction which is shown in figure 1.
To obtain the cyclopentadiene, thermal cracking of the dimer was done using a distillation set-up. The thermal cracking reaction to obtain cyclopentadiene is shown in figure 2. The thermal cracking was done using a Vigreaux column, which is a type of fractionating column used to separate vapours according to their volatility or boiling point. The column consists of a series of downward-pointing indentations to increase the surface area available for repeated condensation and evaporation of the vapours in the column. A temperature of 170 – 190oC was used to heat up the dicyclopentadiene upon which it forms the cyclopentadiene. This cyclopentadiene was collected as the distillate as it is less volatile than the dimer. The temperature at the top of the column registers the boiling point of the cyclopentadiene which is around 39oC. The cyclopentadiene is condensed and collected as the distillate, kept cool in an ice bath.
B: Synthesis of Ferrocene Part (a) – Preparation of potassium cyclopentadienide solution
The cyclopentadienyl solution is prepared by reacting potassium hydroxide, KOH, with cyclopentadiene in anhydrous 1,2-dimethoxyethane (DME). DME was used as a solvent because it is an aprotic polar solvent which allows the dissociation of KOH into K+ and OH- ions. Aprotic solvents like DME solvate the cations but do not solvate the anions. Hence, as the anion OH- is not solvated or stabilized, it can react very easily and deprotonate the cyclopentadiene to form the cyclopentadienyl anion. KOH is hygroscopic hence it should be added quickly to the DME solvent to prevent the absorption of water vapour from the air, which would lead to the formation of KOH solution which is immiscible with the organic layer made up of cyclopentadiene. Protic solvents like water are not used as the hydrogen-bonding interactions with OH- would lead to a decreased interaction with the anion and the cyclopentadiene molecules. Protic solvents reduce the extent of the deprotonation reactions to form the burgundy-coloured (purplish red) cyclopentadienyl anion. Formation of this anion is noticed as the colour of the solution turned deep burgundy, seemingly black. The darker colour observed was due to some cyclopentadienyl anion having been oxidized by O2 that was present. The formation of cyclopentadienyl anion is depicted in figure 3.
The whole set-up is connected to the N2 supply to maintain an inert environment for the preparation of ferrocene. The iron(II) chloride solution to be added in later is susceptible to oxidation by O2 in the air, hence the need to maintain an inert atmosphere. Oxidation of the Fe2+ ion to Fe3+ in iron(II) chloride would only reduce the percentage yield of ferrocene formed. The septum is pierced with an empty needle for 3 mins to relieve pressure in the reaction mixture before cyclopentadiene was added. If cyclopentadiene was abruptly injected into the reaction mixture would lead to result in a pressure drop that causes the mineral oil from the bubbler to be sucked into the reaction mixture, leading to contamination.
B: Synthesis of Ferrocene Part (b) – Preparation of iron(II) chloride solution
In the preparation of FeCl2 solution, FeCl2.4H2O was dissolved in anhydrous dimethylsulfoxide (DMSO). DMSO is an aprotic polar solvent which does not hinder the action of OH- ions in any way. Hence, the deprotonation of cyclopentadiene to form cyclopentadienyl anion would not be hindered after the iron(II) solution is added to the reaction mixture in the round-bottom flask. Furthermore, DMSO is miscible with DME and thus, the Fe2+ ions in solution can interact with the cyclopentadienyl anion to form the ferrocene. Dissolving the FeCl2.4H2O in DMSO made the solution turn light green then it slowly became yellow then orange. This was because there was already some O2 present in the air which caused some of the Fe2+ ions to readily oxidize to Fe3+ ions. This solution was quickly transferred to the dropping funnel to minimise exposure to oxygen in the air. This FeCl2 solution was added dropwise to the round-bottom flask containing the cyclopentadienyl anion to ensure that at any point in time, there is an excess of cyclopentadienyl available to interact with the Fe2+ ion. Furthermore, the mixture was stirred for an additional 30 minutes after all the FeCl2 solution was added to ensure a more complete reaction. These steps ensure a higher yield of the ferrocene product.
B: Synthesis of Ferrocene Part (c) – Formation of ferrocene
In the work-up step, the reaction mixture was added to a beaker containing HCl in ice. The HCl used was to react with the excess KOH in an acid-base neutralization reaction. This prevents the formation of side products like Fe(OH)2 and Fe(OH)3 because the OH- ions could react with the Fe2+ and Fe3+ ions. The ice added was to cool the reaction because the acid-base neutralization is highly exothermic and might cause the decomposition of substances in the reaction mixture. Ferrocene is insoluble in water, and thus it forms an orange precipitate that was filtered off using suction filtration. The crude product was then dried before carrying out the sublimation. The drying process was aided by warming the ferrocene on a hot plate at a low setting to remove any traces of moisture still present in the ferrocene.
For sublimation, the hot plate was turned to a temperature of about 150oC. The crude product that was spread evenly on a Petri dish started to sublime and stick to the other Petri dish that was placed on top. A water-ice mixture in a beaker was used to cool the top Petri dish to aid in sublimation of the ferrocene crystals. Ferrocene sublimes readily under atmospheric conditions because it has significant vapour pressure at temperatures above 100oC. After sublimation, impurities are left behind on the bottom Petri dish. Sublimation is the preferred purification technique because no solvent needs to be added and thus, the product is not contaminated by the solvent. However, it was necessary to heat the crude product gently to prevent overheating or scorching of the product.
IR Spectrum Analysis
IR spectroscopy was carried out on the purified product. IR spectroscopy measures the change in dipole moment of bonds within a molecule as it is subjected to IR radiation. The spectrum that results shows the vibrational modes associated with the molecule itself and indicates the functional groups present in the molecule. This enables the molecule synthesized to be identified as well. The tabulation of assignments to the bands in the IR spectrum is in the results section of this report.
From the assignments table, it could be observed that both the literature and actual spectrum show significant similarities in the peaks present and their associated wavenumbers. The spectrum shows that ferrocene was indeed synthesized. The O-H peak in the spectrum was likely due to some traces of water present in the KBr disc containing the sample.
The IR spectrum also shows the bonding of the cyclopentadienyl ring to the Fe centre via the Fe – Cp stretch. The Cp ring is not bonded to the Fe through any of the carbon atoms but rather, through the -orbital of the ring. The cyclopentadienyl anion formally has 6 electrons whereby the -orbital of the ring involves all 5 carbons in the Cp ring. Furthermore, the +2 charge on the Fe2+ ion balances the -1 charge on each of the two Cp rings. The presence of sp2 C-H stretching and C=C stretching confirms that the cyclopentadienyl ring is still intact and not modified by the bonding to the Fe2+ centre. Aromatic OOP bending and overtones detected in the spectrum reinforce the face that the cyclopentadienyl rings are still aromatic in ferrocene. There is also the cyclopentadienyl tilting which gives rise to a peak in itself, thus all these results show that the ring retains its structure and aromaticity in ferrocene.
With regards to the structure, the sandwich structure has the Fe2+ situated in-between two cyclopentadienyl rings. The cyclopentadienyl rings can take an eclipsed (D5h), staggered (D5d) conformation or anything in-between in ferrocene as shown in figure 4. The energy of rotation about the Fe-Cp axis is very small (~ 4 kJ mol-1) and ground state structures of ferrocene may show either of these conformations. Ferrocene is an 18-electron species whereby the Fe2+ of d6-configuration contributes 6 valence electrons from its while each of the Cp rings contributes 6 electrons as well. Also, the Fe centre is sterically protected by the Cp rings. These factors give rise to the stability of the complex up to 400oC.
MO Diagram of Ferrocene
There is very little difference in the electronic states of D5d and D5h for the conformation of ferrocene. Thus D5d has been used as the point group representation in the description of the electronic structure for ferrocene as it simplifies the symmetry matching of the ligand molecular orbitals and the metal atomic orbitals. In ferrocene, the primary orbital interactions that form the metal-ligand bonds occur between the Fe s, p and d orbitals with the -orbitals of the Cp ligand.
The MO diagram shows the symmetry-adapted linear combination of molecular orbitals (SALCs) arising from the-molecular orbitals of the cyclopentadienyl rings and the overlap with the corresponding atomic orbitals of Fe. For the planar Cp ring (D5h symmetry), the five p-orbitals on it can be combined to produce five -molecular orbitals. These five -molecular orbitals consist of the a2 bonding (0 nodes), two e1 weakly bonding (1 node) and two e2 anti-bonding (2 nodes) molecular orbitals. Only the a2 and e1 MOs are filled with the 6 -electrons from the cyclopentadienyl ring. The -orbitals of the two Cp ligands in the ferrocene molecule are then combined pairwise to form the SALCs and this gives rise to the following orbitals of gerade (g) and ungerade (u) symmetry with respect to the centre of inversion: a low lying filled bonding pair of a1g and a2u symmetry, a filled weakly bonding pair of e1g and e1u symmetry and an unfilled anti-bonding pair of e2g and e2u symmetry. These SALCs of the pair of cyclopentadienyl rings have been displayed on the left side of the MO diagram in figure 5. These ligand molecular orbitals are then considered with respect to the metal orbitals and whether or not there is symmetry-allowed orbital overlap. This enables the MO diagram of ferrocene to be constructed.
These types of overlaps are shown in the above figure 6 which shows the different types of bonding (, and ) occurring in the ferrocene compound. Fe2+ has atomic orbitals of 4s (a1g), 4pz (a2u), 3dz2 (a1g), 3dxz and 3dyz (e1g), 4px and 4py (e1u) as well as 3dx2-y2 and 3dxy (e2g). These atomic orbitals overlap correspondingly with the molecular orbitals of the cyclopentadienyl rings. It could be observed that the HOMO orbitals of the ferrocene lie close to the d-orbitals of the Fe2+ ion, and hence the bonding that holds the cyclopentadienyl rings to the Fe centre is mainly due to these orbitals which have d-orbital character. This explains why the bonding between the Fe2+ and the cyclopentadienyl ligand does not affect the structure of the cyclopentadienyl ring.
The colour of ferrocene is golden-orange as reported by some literature texts. The UV-vis spectra for ferrocene shows maxima at 330 nm ( = 52 M-1 cm-1) and 440 nm ( = 90 M-1 cm-1) as well as a rising short-wavelength absorption at 225 nm ( = 5051 M-1 cm-1). The absorptions at 330 nm and 440 nm are probably Laporte-forbidden d-d transitions which occur within the HOMO-LUMO energy gap. From the MO diagram, these transitions of 330 nm and 440 nm arise from e2g → e*1g and a’1g → e*1g respectively. Meanwhile, for the transition at 225 nm, this is a charge-transfer transition arising from the e1u → e*1g transition which is both spin and Laporte allowed. Hence, it has a higher molar absorptivity than the d-d transitions. This CT is from an e1u orbital which is more ligand in nature to an e*1g orbital that is more metal in nature, hence this would be classified as an Ligand-Metal Charge Transfer (LMCT). The golden-orange colour of the ferrocene would be due to the absorption in the 440 nm, which the human eye can detect. The absorption of blue colour at 440 nm gives rise to the complementary golden-orange colour of ferrocene.
Yield of Ferrocene
The yield of ferrocene for this experiment is only 32.7% and this is considerably low as compared to the literature yield of 93%. This could be due to the KOH having absorbed water due to its hygroscopicity before it was mixed with the cyclopentadiene. Furthermore, the KOH came in pellets, which made it more difficult for it to ionize in DME. To ensure a better yield, the KOH should be stirred in with the DME for a longer time to ensure it forms sufficient amounts of potassium cyclopentadienide for reaction. Also, the Fe2+ ion in DMSO was oxidized too readily by oxygen present in the 25 ml conical flask. This led to a decrease in the Fe2+ ions available for reaction to form ferrocene. Perhaps the conical flask should have been filled with N2 gas and stoppered before placing the FeCl2.4H2O in it. This would reduce the likelihood of Fe2+ oxidising. The experimental set-up could also have been filled with nitrogen for a longer duration to ensure a more inert environment for the reaction. This is to prevent oxidation of the cyclopentadienyl and the Fe2+ that are reacting in the round-bottom flask.
Melting Point of Ferrocene
The melting point of ferrocene obtained is 172.80 – 173.90 oC and this was close to the literature melting point of 173 – 174 oC. This shows that the ferrocene obtained was indeed pure due to the efficacy of the sublimation process. The close resemblance of the IR spectrum with that of literature provides further evidence of the purity of the synthesized ferrocene.
Conclusion
Ferrocene was synthesized from cyclopentadiene and iron(II) chloride under basic conditions with a yield of 32.7%. The melting point of ferrocene was determined to be 172.80 – 173.90 oC.
Exercises
1. (a) Assignment of peaks for 1H NMR of Ferrocene
/ ppm
|
Multiplicity
|
Assignment
| |
1.5
|
Singlet
|
H2O
| |
4.1
|
Singlet
|
H (in ferrocene)
| |
7.24
|
Singlet
|
CHCl3
|
The peak at 4.1 ppm belongs to the protons in ferrocene. Due to the five-fold symmetry of the cyclopentadienyl rings, the protons are all chemically equivalent, hence there is only one signal. The rapid rotation of the rings around the Fe centre results in the rapid equilibration of the protons in ferrocene. Furthermore, there are no neighbouring protons to couple with thus the signal is a singlet. The chemical shift value of 4.1 ppm is lower than that for aromatic protons at 7 ppm because there is an increased electron density in the two rings that increases the shielding effect, causing a decrease in the chemical shift value.
Meanwhile, the other two peaks in the spectrum at 1.5 ppm and 7.24 ppm are due to H2O and CHCl3. The solvent used in the NMR is CDCl3 but this solvent is not entirely deuterated, there is still a small percentage of solvent present as CHCl3 which gives a characteristic peak at 7.24 ppm. The other peaks in the spectrum are calibrated with respect to this reference signal. The peak at 1.50 ppm is due to H2O present which means that the ferrocene is not completely dry and there were traces of water present in the sample tested for NMR. The water present presumably comes from water vapour condensing on the product during the sublimation process.
1. (b) Assignment of peaks for 1H NMR of Cyclopentadiene (low temp)
/ ppm
|
Multiplicity
|
Assignment
| |
3.95
|
Triplet
|
Ha
| |
5.54
|
Multiplet
|
Hb, Hb’
| |
6.00
|
Multiplet
|
Hc, Hc’
|
For cyclopentadiene, there are three signals due to the three separate chemical environments of the protons. The signal at 3.95 ppm is assigned to Ha which is furthest away from the C=C and hence, experiences lower deshielding effect due to the anisotropic effect of the C=C double bonds. The chemical shift is still higher than that for a normal sp3 hydrogen (~ 1-2 ppm) as there is some anisotropic effect experienced due to the C=C double bonds. Hb and Hc are directly bonded to the C=C double bonds and hence directly experiences the anisotropic effect, and this causes the protons to become more deshielded. This leads to higher chemical shift values for these peaks. Hc is more deshielded than Hb because besides being directly bonded to one C=C, it is closer to the other C=C as well thus leading to a greater deshielding effect.
The protons in ferrocene have a chemical shift of 4.1 ppm which is higher than that due to the Ha protons in cyclopentadiene. This indirectly points to the aromatic system of the cyclopentadienyl rings in ferrocene which creates an average double bond character in the ring leading to the anisotropic effect causing a slightly greater chemical shift value of 4.1 ppm as compared to the 3.95 ppm of Ha in cyclopentadiene. However, this value of 4.1 ppm is less than that due to Hb and Hc of cyclopentadiene as the double bond character of the cyclopentadienyl ring in ferrocene is weaker than a normal C=C double bond because the -electrons are spread over the five carbon atoms.
The protons in cyclopentadiene are not chemically equivalent, hence they do couple with each other. For Ha, it is a triplet because it couples with the two neighbouring Hb and Hb’ protons. However, this is not a simple first-order triplet. Rather, there are second-order effects seen as the Hb proton is not magnetically equivalent to the Hb’ proton. This is due to the non-planarity of the cyclopentadiene and whether or not the protons attached to the double bonds are in cis or trans conformation. Second-order effects are also observed for the other protons. Hb should couple with Ha and Hc to give a doublet of triplets but this is complicated by further coupling to Hb’ and hence, a multiplet is formed instead. Similarly, for Hc, it should couple to Hb to give a doublet, but it also actually couples to the other Hc’ and hence a multiplet is observed. Hc and Hc’ are symmetry equivalent but not magnetically equivalent, as are Hb and Hb’. Magnetically inequivalent nuclei have different coupling constants with respect to other nuclei.
2. Ferrocene might be stable to air and moisture but its constituent reactant molecules of cyclopentadienyl and Fe2+ are susceptible to oxidation by oxygen present in air. Thus, an inert environment would be required to ensure the maximum possible yield of ferrocene from the reactants. The set-up should also be dry because moisture present would react with the hygroscopic KOH and reduce the amount of OH- ions present to deprotonate cyclopentadiene. Water has a pKa of 14.0 whereas cyclopentadiene has a pKa of 15.5. Thus the OH- ions would prefer to deprotonate water as it has a lower pKa value. This means less cyclopentadiene would be present as the potassium cyclopentadienide required for reaction. This again, would reduce the yield of ferrocene.
3. Cyclopentadiene dimerizes readily at room temperature by a Diels-Alder reaction to form dicyclopentadiene. Leaving cyclopentadiene for long periods of time might also lead to increased dimerization even if it is stored at low temperatures. Thus, it is best to freshly prepare the cyclopentadiene just before it is used to synthesize ferrocene.
4. The apparatus used should be washed with acetone and dried in the oven before use. The set-up should be flushed with N2 gas for a short duration of about 10 minutes to create the necessary inert environment for the reaction before any other steps are carried out. Perhaps even specialised apparatus like nitrogen dry boxes could be used in preparation of the reagents in the experiment. The nitrogen dry box is an enclosed chamber that maintains a dry and inert environment for the experiment.
References
5.8 Symmetry in NMR Spectra. University of Wisconsin. Available at: http://www.chem.wisc.edu/areas/reich/nmr/05-hmr-08-symmetry.htm. Last accessed 12 June 2011.
B.V. Lokshin, V.T. Aleksanian and E.B. Rusach. On The Vibrational Assignments of Ferrocene, Ruthenocene And Osmocene. Journal of Organometallic Chemistry, 86 (1975) 253 – 256.
Experiment 7: Friedel-Crafts Acetylation Chemistry. Pg 63 – 65. The University of Oxford. Weblearn Content. Available at: http://weblearn.ox.ac.uk/site/mathsphys/chem/prac/dp_lab/dp_lab_y2/Experiment%207%20-%20Friedel-Crafts.pdf. Last accessed 12 June 2011.
Pamela S. Tanner, Gennady Dantsin, Stephen M. Gross, Alistair J. Lees, Clifford E. Myers, M. Stanley Whittingham and Wayne E. Jones, Jr. [1]. The Synthesis and Characterization of Ferrocene. A Modern Iterative Approach to a Classical Organometallic Laboratory Experiment. State University of New York at Binghamton, Binghamton, New York.
J. N. Willis, JR., M. T. Ryan, F. L. Hedberg and H. Rosenberg. An infrared and Raman study of l,l’-disubstituted ferrocene compounds. Spectrochimica Acta,Vol. 24A, pp. 1561 to 1572. Pergamon Press 1968.
Nice
ReplyDeleteWhere did you find these Lit values the IR of ferrocene?
ReplyDeleteNo. of moles of KOH used: 4.0081 / 56.11 = 71.43 mmol
ReplyDeleteThe mole ratio of C5H6 to KOH to FeCl2.4H2O is 2:8:1. As 11.889 mmol of C5H6 required 47.556 mmol of KOH
why does moles change?
nvm i see
DeleteI need an explanation please
Deleteincorrect
ReplyDeleteHi
ReplyDeleteCould you provide a reference for the paragraph in which you discuss the colour of ferrocene.
Ai Furnaces is a world-class manufacturer of lab furnaces and ovens .Our high-quality furnaces are built to use in the most demanding conditions. 100% satisfaction guaranteed.
ReplyDeletelucciOnue_pi_Everett Jose Hawkins https://marketplace.visualstudio.com/items?itemName=quoculnasde.Odd--Even-gratuita
ReplyDeletegeolocyzo
WinmenVel-no Stacy Brown https://www.herefordrc.co.uk/profile/HD-Online-Player-gintama-Movie-Shiroyasha-Koutan-Down-UPDATED/profile
ReplyDeletepenramangcard
Ostinperlio_ku Michael Kishore Check
ReplyDeleteInstall
download now
https://exe2zip.com/eset-nod32-smart-security-download-full-nulled-serial-number/
lialimucksan